WEI-LUN HSU
PhD
Concentration Gradient Focusing of proteins in a silica nanofluidic channel containing a varying electroosmotic flow is analysed experimentally and theoretically. The trap locations of the proteins depend on their charge to size ratio. The larger sized (lower diffusivity) and lower charged proteins are trapped closer to the low salt concentration end. The simulations quantitatively capture the observed trapping behaviour of a yellow/red fluorescent protein (R-PE). Analysis suggests that protein trapping becomes saturated when the electric double layers on the proteins overlap. Protein trapping conducted in a nanochannel which has a larger channel width at the low salt concentration end has a lower CE, peak located closer to the low concentration end and wider peak width. We find that the electroosmotic behaviour caused by a concentration gradient in a silica nanochannel is different from previously described: the nonuniform electric field and electric double layer along the nanochannel surface (due to the concentration gradient) induces a counter-electroosmotic flow near the nanochannel centreline at the low salt concentration end. This counter flow enhances the protein trapping efficiency while not hindering the separation ability. The separation of yellow/red fluorescent protein (R-PE) from green fluorescent protein (Dyl-Strep) as previously observed in a similar system is qualitatively captured by the simulations.

Lab-on-a-Chip

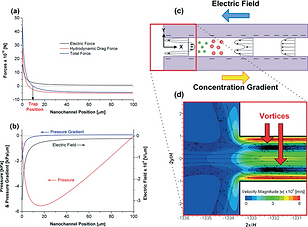
Concentration Gradient Focusing and Separation of Proteins
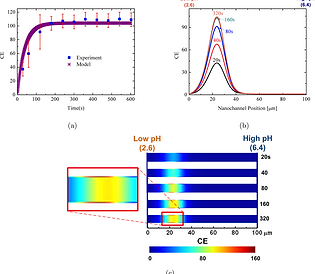
Isoelectric Focusing and Separation of Proteins
Isoelectric focusing of Dyl-Strep is investigated by considering the electrophoretic and electroosmotic behavior of amphoteric molecules in silica nanofluidic channels filled with a controlled gradient of citric acid and disodium orthophosphate buffer solutions. The simulated concentration enhancement (CE) closely matches both transient and steady-state experimental data. The effects of focusing time, pI, applied voltage, bulk pH and bulk conductivity on the traps and pH gradients are examined theoretically. Electromigration drives the traps toward the anode (the low pH end) while electroosmosis pushes the traps toward the cathode (the high pH end). The protein trapping CE increases rapidly in the first 100 s and reaches a steady-state within approximately 3 min. The trap locations are closer to the low pH end at the larger applied electric potential. The CE reaches a maximum value when the trap location is at around 40 μm from the low pH end in the nanochannel. Protein adsorption like behavior is observed on the nanochannel surface near the trap location. The trap location drifts to the high pH end when the pH in the high pH microchannel is lower or the bulk solution conductivity is lower. The electroosmotic velocity profile in the silica nanofluidic channel is nonuniform and a local recirculating flow is formed when the bulk solution conductivity is low.

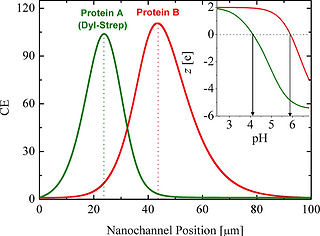